
All cell lines were transiently transfected in order to express fluorescent protein (FP) fusions of marker proteins for the following cellular components: plasma membrane, ER, mitochondria, Golgi apparatus and the nucleus. Having established that mammalian cells can readily increase in size upon exposure to hypotonic solutions, we wanted to look closer at the consequences on intracellular organelle and membrane sizes. Mammalian cells recover from the swelling when replaced in regular culture media, allowing for multiple transient analyses of the same cell(s) over time. We also demonstrate that cell swelling is suitable for live cells. As proof of concept we demonstrate precise determinations of protein locations on endoplasmic reticulum (ER) membranes and lumens, and mitochondria inner/outer membranes and matrixes, all performed within a few minutes on a conventional widefield or confocal microscope at no extra costs. We show that this approach provides a fast and reliable way to determine exact protein sub-locations and organelle quantities that are otherwise very difficult to achieve. Although swelling can be induced similarly in yeast 12 and bacteria spheroplasts 13, we here focus on the many benefits of induced mammalian cell and organelle swelling in hypotonic solutions.
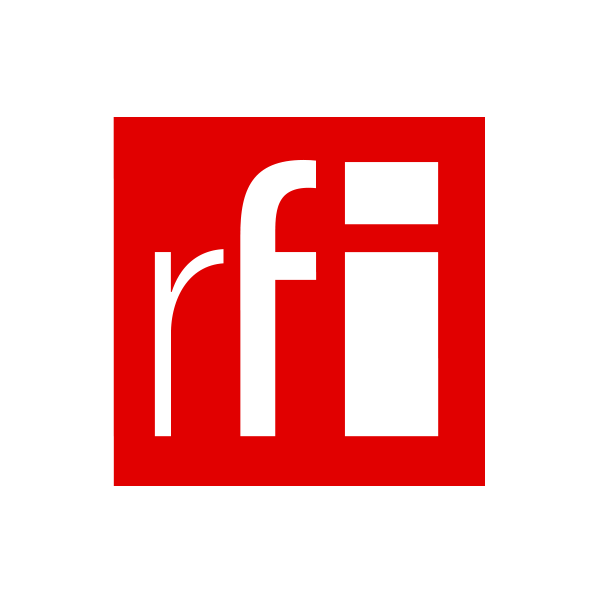
The resulting swelling of the cell and organelles is not isotropic, which can be very beneficial from an imaging point-of-view (Fig. In short, hypotonic solutions cause water influx into the cell’s interior to equal osmotic concentrations. Moreover, both live mammalian cells and many of their organelles can easily be enlarged by exposing them to hypotonic solutions 11. However, live cells have the ability to regulate their volume, which has been extensively reviewed 10. Since expansion microscopy relies on progressive enlargements of fixed biomaterial, its protocol is rather time-consuming (up to several days) and obviously unsuitable for live cells 9. We asked if this idea of modifying the sample rather than the optical instrument could be explored further and extended to live cells. Numerous studies have since shown that expansion microscopy can indeed be successfully applied to cells and tissues 8. Expansion microscopy 7 argues that by isotropically expanding biomaterial, otherwise hidden structural information could be lifted into the resolvable range.

Recently, a new approach for improving spatial resolution has been introduced which puts the focus away from the instrument onto the sample itself. electron microscopy, Förster resonance energy transfer etc.) or have to wait until new and often expensive technological advances provide solutions. If biostructures are too small for a given optical instrument to resolve, scientists can either try to find answers by using related technologies (e.g.
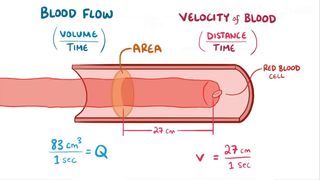
In any case, whether a certain biological structure is resolvable or not depends on two separable factors: the resolution limit of the chosen optical instrument and the size of the biological structure. Especially superresolution light microscopy is able to provide such data from fixed samples and, at often lower spatial resolution, from live cells 1, 2, 3, 4, 5, 6. This puts a technical challenge on bioimaging since many organelles’ membrane assemblies are well below the micrometer range in size and distance. More precise information is needed, for example whether the protein is membrane-bound, or not. To know that a certain protein is located within a particular organelle is often not sufficient. Especially in the spatio-temporal context of cellular dynamics the precise protein sub-location within the membranous organelle system of the cell is crucial. Besides the mere metrics of biomaterial like cells, organelles, or even sub-organelle structures, more informative are data addressing protein sub-locations and interactions. Scientific bioimaging aims to reveal scientifically relevant information.
